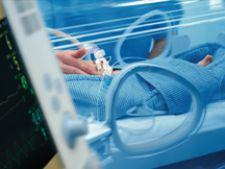
Christer Sinderby has conducted extensive research within neurally controlled ventilation.
Intensive Care Units around the world have been providing their patients with mechanical ventilation for the past 30 years, ever since the first electronic ventilator technology appeared in the early seventies. And ventilation therapy, irregardless of mode, has been delivered in the same manner — a clinician has regulated the pressures and volumes provided to the patient.
A group of researchers in Toronto has established a new respiratory methodology, based on the neural signals provided by the patient. Research in the concept of Neurally Adjusted Ventilator Assist (NAVA) is rapidly gaining the interest of the scientific community.
Can you describe to our readers what NAVA (Neurally Adjusted Ventilatory Assist) is, and how it functions?
NAVA is a new mode of mechanical ventilation, where the ventilator is controlled directly by the patient's own neural control of breathing. Since the introduction of the mechanical ventilator over thirty years ago, ventilation treatment has traditionally been provided by means of adjusting airway pressure, flow and volume. With NAVA, the ventilator pressure is adjusted by the patient's own neural control system. The neural control of respiration originates in the respiratory center, and signals are transmitted through the phrenic nerve to excite the diaphragm.
With NAVA, these signals are monitored by means of bipolar electrodes mounted on a nasogastric feeding tube and positioned in the esophagus at the level of the diaphragm. As respiration increases and the respiratory center requires the diaphragm for more effort, the degree of NAVA ventilatory support needed is immediately provided. This means that the patient's respiratory center is in direct control of the mechanical support required on a breath-by-breath basis, and any variation in the neural respiratory demand is responded to by the appropriate corresponding change in ventilatory assistance.
Besides being a distinct mode of ventilation, NAVA also offers a complete evaluation of the neural respiratory control in the context of a respiratory monitor. For example, if no assist is provided, the drive will be very large, but if the patient is provided with assist, the drive decreases. These are rapid reflex loops that are constantly ongoing. This provides both the neural monitoring signal and how much assist is to be delivered, at the same time. It also shows the extent of how the respiratory drive has been regulated, and the pressures used in this respect.
There are no other modes that can provide these capabilities today. A pulse oximeter may tell us: “The saturation is going down to 80 percent and affecting PaO2, and if FiO2is increased, the saturation increases back up to 91 percent.” But that is only a monitor, and the clinician is in charge of making the required adjustments. With NAVA, the patient is “in charge” of adjusting the ventilation.
We do not make any interventions; only observe how the patient is regulating himself.
One interesting aspect is in emergency situations; we have found that it is always safest when we apply NAVA. We seem to have much better control than by any other means. In fact, we have found that the sicker the patient is, the better NAVA seems to respond.
Right now in Toronto, we are studying heavily sedated patients breathing on NAVA, with very few difficulties, blood gases are normal and the patients are stable. This is of special interest, since it is a new area of research.
What led to your research in this particular area, and how did you establish this concept?
It started with signal analysis of electrical signals from the diaphragm, a field where we had been doing research for many years. We started with something called spectral analysis, which is examining the diaphragm electrical activity in the frequency domain. The frequency domain can provide a great deal of information, for example when a muscle is fatigued, the number of motor units a muscle has, and how the muscle is recruited, which is the signal used when triggering breaths.
We plotted the frequency spectrum to 1024 different points, and divided the points to evaluate the signal, so we made an enormous analysis of every little sample. This in turn led to a practical solution, which a good friend of mine, Dr Paolo Navalesi, an intensive care physician, asked if we were interested in using this technology for evaluating ventilators. By this time, we were doing research on respiratory muscle fatigue during weaning. We went ahead and evaluated the signals we had, and observed that the signals were very stable, and that there were significant delays with triggering with conventional ventilators, which made us think that we could do a much better job.
So from that point on, with the signal processing research, which we had already developed, it was not very complicated to obtain algorithms for controlling the ventilator. The difficulties arise when attempting to apply these algorithms into current ventilator technology and existing platforms, where adaptations to already existing technology must be considered.
Will the biggest challenge with NAVA be that people will have to regard respiratory therapy in a fundamentally different and new way?
Traditionally, many physicians who have worked in the ICU have had a background as an anesthesiologist or an intensivist. With NAVA, this means that they may have to think in terms of neurology as well. But a neurologist typically doesn't think in terms of respiratory therapy, either.
But the potential benefits may outweigh these challenges, for example when we see neonatal patients being treated with nasal ventilation, and the CT scans confirming that their lungs are completely recruited.
It is not a matter of one group being able to do something a little better than another; rather that we are opening a completely new door. It is a significant paradigm shift, which may offer opportunities to do things we never felt possible before. But this requires a totally new manner of thinking.
When we have the opportunity of lecturing to ICU staff, we try to communicate that this is new, this is pioneering, and we may be able to do things that were not possible in the past. But an entire group of professionals will have to start considering this new generation of mechanical ventilation as well, which is necessary with the introduction of a new methodology.
There has been a growing interest in asynchrony in recent years, however it is still perhaps not prioritized as it might be in daily treatment work.
From your perspective, how frequently does patient-ventilator asynchrony occur?
I believe that there is a level of patient ventilator asynchrony in every patient, and in every mode of pneumatically-driven ventilation. From my experience, it is rare to find patients with insufficient support. In most cases, patients are receiving too much support, and this means that the assist is prolonged beyond the neural cycle. In this situation, triggering the ventilator can become a problem for certain patient groups, for example COPD. But knowing when to terminate inspiration — there are no physiological cycling-off criteria available today.
We have previously observed and shown that asynchrony occurs in intubated adult patients with mixed etiology (Beck et al, 2001, Spahija et al, 2005) and in intubated infants being weaned from the ventilator (Beck et al 2004). In particular, by having the ability to monitor the neural activity of the diaphragm during mechanical ventilation, opens the possibility to evaluate patient-ventilator asynchrony at the bedside. Ventilator asynchrony can be compared to other technologies: who wants a car that has a five second delayed reaction when you accelerate, and sometimes brakes, and sometimes does not brake at all?
Or a computer mouse that reacts long after you have clicked on it,or with lightening speed so you can not catch up at all?
Exactly — this is basic supply and demand. A methodology and system should deliver a support in the amount and time that the patient needs it, which allows the level of support to decrease over time. This is the support we are striving to obtain, which is why synchrony is important.
In terms of patient risks in connection with asynchrony, what do you believe is most significant?
One of the physiological effects of patient ventilator asynchrony is that it interferes with the patient's natural breathing pattern. As we have shown in intubated infants, excessive delivery of assist (beyond the neural inspiration) prolongs expiration as compared to an unassisted breath (Beck et al 2004). In addition, if there is a delay to trigger the ventilator, this will prolong inspiratory time. With NAVA, the ventilator is cycled on when neural inspiration begins, it provides assist during inspiration proportionally, and it cycles off when the neural expiration begins. It does not interfere with the natural breathing pattern, because it follows the natural breathing pattern.
One of the greatest problems arising from excessive delivery of assist (delayed cycling off) is that extreme pressures and volumes can cause lung injury. Our most recent experience in experimental research suggests that NAVA is lung protective (Brander et al, 2006). Our clinical data also indicates the potential for lung protection as the spontaneously chosen tidal volumes are low and similar to those recommended by the ARDSNet study for reducing ventilator-induced lung injury. In three pre-clinical studies and two human studies, we have tried to increase the NAVA assist to levels comparable to overdistention. And we have not been able to come up to these levels — there is a neurological reflex that regulates and closes pressures that are too high.
Physiologically, when a volume increases to a certain level where you can no longer inspire, the body has a neurological mechanism that stops the process. This is a fundamental difference from mechanical ventilation, where a third party regulates volumes and pressures. But synchrony is two-dimensional: a time scale where it might be too late to start to inspire or too early or too late to cycle off, and the other dimension is amplitude.
But the protective component is not only in amplitude. For example, when we have studied neurally triggered pressure support, and deliver high pressure support levels, in these cases the patient time scale cycles off so early that it is not possible to go over a certain level. If the volume is obtained too rapidly, it stops, from a neural perspective. This is the same pattern of normal respiration; you cannot inspire above a certain level that the body does not accept.
With NAVA, a ventilator would function in the same manner as the body in this case — you cannot prolong a breath. If a breath were prolonged, there would be excessive volume, resulting in lung injury.
So NAVA covers both dimensions, the time scale and the amplitude. And the benefit is that you have a methodology that does not lead to a run away situation, but allows the body's physiological defense mechanism to regulate the ventilator.
General asynchrony can be compared to an example: if a patient tries to breathe and get insufficient support, the inspiration is insufficient. If the ventilator then should start when the patient has stopped inspiring, they receive the wrong level of assist and become agitated. If I thereafter increase the support from the ventilator to supply larger pressures, the patient might miss a few breaths, and we have initiated the downward spiral.
If patient-ventilator asynchrony is so severe that the patient “fights” the ventilator, the caregiver usually resorts to sedation and or neuromuscular paralysis of the patient. However, the current trend in mechanical ventilation is to give less sedation and to help the patient breathe spontaneously. If we consider spontaneous breathing to be a true objective in ventilation therapy, we must help the patient to receive a level of support that is adapted to spontaneous breathing, and interfere as little as possible.
Respiratory support should only be delivered when the patient wants it. Traditionally, it has been a matter of supplying a ventilation support that sustains a certain level of minute ventilation, but the ventilator must take care of the lung, chest wall and abdomen, and the work of breathing increases. The patient does not receive what he needs.
What considerations should be taken with NAVA in regard to intubated as well as noninvasively ventilated patients?
If you are treating an intubated patient, you have a patient who has lost some level of autonomous breathing control functions (you have blocked the upper airway defense mechanisms). Perhaps you want to protect the airways if the patient is very sedated. With neural control, it does not matter how you ventilate; we can ventilate with a mask, with a helmet, nasal prongs, a mouthpiece or an endotracheal tube. We see the same results however we deliver ventilation; the fact that we are delivering neurally controlled ventilation is not an issue. However, this difference between invasive versus non-invasive is a traditional way of thinking in regard to delivery of ventilation therapy.
But for example, some patients must be sedated, if they are in pain or very ill, and NAVA can still support ventilation in these cases. NAVA delivers the support when the patient neural signals demands a breath, and NAVA delivers support that is proportional to the patient's neural request, and stops when the patient has received enough. It works if the patient is intubated or ventilation is delivered by other means.
This is a matter of disconnecting pneumatics in how we consider this: the tube, whether it is in the nose or in the throat, is only there to deliver ventilation. Today, physicians use pressure and flow as feedback for supplying support, which means a lot of information is needed from the same tube. With NAVA, we take the information from another source, directly from the brain, if you will, and that information comes directly outside any other routes.
Are any particular patient categories of special interest in terms of NAVA, at this point in time?
In regard to patient categories, I believe it is very important to gain experience in as many types of intensive care patients and disease categories as possible. Heart-lung transplant patients have many organs where the neural connection has been cut and replaced by new organs, and I do not think they would be good candidates for NAVA ventilation at this stage; it is perhaps too early yet.
In regard to other different patient categories — we are in the pioneering stages. We are entering into a new generation in mechanical ventilation. For myself as a researcher and for the team I work with, we are doing as much as we can to obtain as much good information as we can at this stage.
Our total focus right now is on safety. We hope that other intensivists and research centers with a pioneering spirit will want to join us in the development of a new methodology, something fundamentally different than everyone is used to.
There is an exciting journey ahead of us, with experiences that we may have not expected, but also groundbreaking therapeutic discoveries in the near future. This will require an enormous amount of communication, and forums for clinicians to share experiences with each other.
When we try something new for the first time, it may easily happen that we experience that it does not work according to our expectations, which means it is a question of diagnostics and translation, in the process of learning the patterns of treatment with a new methodology. What is important to note is that the initial physicians working with this will be true pioneers. We have a first solution how NAVA can be applied, but we will collectively need to share all of our experiences as we go forward. A new way of thinking and a new perspective on ventilation means not only potentially new solutions, but potentially new challenges as well within the future in critical care.
Christer Sinderby, Ph.D. is assistant professor at the Department of Medicine, University of Toronto, Canada, and research staff scientist at St. Michael's Hospital, Department of Critical Care Medicine, Toronto.
Reprinted with permission from Critical Care News, Maquet Critical Care