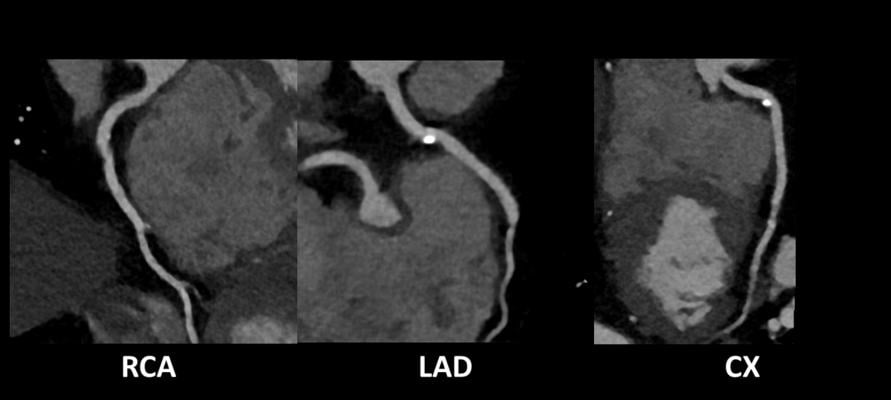
RCA – Right Coronary Artery; LAD – Left Anterior Descending; CX – Circumflex Artery. Image credits: Case courtesy of Joachim Feger, https://radiopaedia.org/articles/cardiac-ct-1
Coronary artery disease (CAD), also known as ischemic heart disease, is the most common heart disease with a prevalence in the United States of approximately 7% in people 20 years and older (1). CAD is caused by atherosclerotic plaque buildup in the wall of the coronary arteries, characterized by thickening of the arterial intima, and is typically composed of a lipid core with an overlying fibrous cap. This leads to arterial lumen narrowing and eventually a complete blockage of blood flow to cardiac muscle. Generally, coronary plaques are classified into calcified and non-calcified plaques. The calcified plaque contains water, lipid, protein, and calcium while the non-calcified plaque contains only water, lipid, and protein. Clinically, CAD is diagnosed and monitored through different tests, but angiography remains the gold standard for diagnosis and quantification of atherosclerotic plaques.
Clinical CAD imaging is based on angiography; however, coronary computed tomography angiography (CCTA) has more recently been positioned as the primary diagnostic imaging modality. Angiography allows for understanding the presence and anatomical severity of CAD, but with CCTA characterizing the anatomical structure and physiological impacts of the CAD-associated plaques are possible. Computed tomography visualizes the three-dimensional anatomical geometry, as well as discern changes in tissue density, depicted in Hounsfield units (HU). The HU variations across tissue types makes CCTA capable of differentiating between coronary artery plaque types and features, which is beneficial for determining risks associates with CAD.
Considerations for CCTA image acquisition
As CCTA progresses towards becoming the primary imaging modality for not only diagnostic purposes, but also for clinical trial endpoints, there is a need to address the requirements for generating high-quality CCTA image data to ensure accurate and robust CAD assessments. The aim here is to introduce considerations for CCTA image acquisition, and anatomical and functional imaging-based assessments in atherosclerotic cardiovascular disease (ASCVD) clinical trials.
Technical requirements and guidelines have been formulated and standardized within the field (2, 3) which provide the basis for the minimum hardware requirements, acquisition parameters, subject preparation considerations, and data post-processing to ensure high-quality images are acquired and suitable for diagnostic standards and applicable quantitative analyses. When discussing image quality, the key imaging requirements are minimizing the dynamic motion of the heart to prevent motion artifacts and obtaining high contrast between the coronary arteries and surrounding cardiac tissues.
Steps to improve image quality begin with subject preparation actions and set the goal of minimizing heart rate to reduce the risk of motion artifacts. The typical target for CCTA imaging is about 60 beats per minute, however, with modern scanners, some imaging protocols may achieve successful imaging with higher heart rates. By reducing heart rate, image quality is improved by acquiring during specific cardiac phases and opens the possibility to using radiation dose reduction protocols. Steps to control heart rate and cardiac rhythm may be taken, including the use of beta-blockers ranging from pre-medication up to 24 hours in advance to oral dosing 1 hour prior to exam and additional IV dosing immediately before CT scan acquisition.
Coronary artery vasodilation aids to improve image quality, by enlarging the arterial lumen via vascular smooth muscle relaxation. Stimulating vasodilation has been demonstrated to improve coronary artery visualization and stenosis assessment. Vasodilation is effectively achieved by using nitrates and should be administered shortly prior to the scan acquisition to ensure the maximum vasodilation response is captured during the CCTA imaging.
To further reduce motion artifacts caused by the surrounding lung tissues during image acquisition, it is recommended for acquisition during an inspiratory breath hold. The subject should practice a breath hold prior to scan acquisition to observe the quality of the subject’s breath hold, ensure adequate breath hold length of time, and identify any fluctuations and variability of heart rate and rhythm associated with the breath hold. Breathing introduces significant motion artifacts precluding any qualitative or quantitative assessments, so it is recommended to not proceed with imaging if a breath hold is not achievable.
The contrast injection protocol is crucial to obtaining a high signal-to-noise ratio during the scan acquisition, as the contrast medium provides the arterial lumen enhancement to visualize the coronary arteries. Optimal contrast enhancement can be attained by using a high flow rate to inject the iodinated contrast medium through a catheter. By adjusting the injection protocol, such as a biphasic and triphasic protocol, different cardiac anatomy and features are enhanced for analysis. Scanner manufacturers and institutional standard practices vary, but the goal is to ensure adequate contrast enhancement during image acquisition. Additionally, subject positioning, ECG placement are critical, as these steps directly contribute to the image quality by removing additional anatomy and objects outside the scan acquisition field-of-view, which can introduce noise, beam hardening or metal streak artifacts within the reconstructed images.
Key factors influencing image quality
The key factors influencing image quality are related to the image acquisition parameters, which affect the overall image quality but also need to consider radiation exposure and scan duration. Typically, as radiation exposure increases, the image quality improves; however, it is important to consider the acceptable radiation doses and maintain principles of ALARA (As Low As Reasonably Achievable). Several factors contribute to the radiation dose and image quality, including scanner hardware, acquisition parameters, and image reconstruction post-processing. Imaging protocols should adapt the tube voltage and current to achieve optimal image quality. Tube current modulation techniques, such as ECG-based modulation, should be considered to lower radiation exposure, while image acquisition occurs at the cardiac phase with minimal cardiac motion.
Cardiac gating minimizes radiation exposure while maintaining image quality. The two modes, either prospective or retrospective ECG-triggered gating, are recommended based on heart rate and variability. Prospective gating reduces radiation exposure, but the acquisition is limited to a narrow cardiac phase window, limiting additional cardiac phase reconstructions options. Retrospective ECG-gating may be considered more appropriate if lower heart rate and regular rhythm cannot be achieved. Although retrospective gating costs additional radiation dose, all cardiac phases are captured during the acquisition, so the optimal cardiac phase image with reduced motion and optimal image quality can be generated.
Image reconstruction parameters influence the final reconstructed image as related to image quality/noise, edge definition, resolution, and imaging artifacts. Image reconstruction recommendations are to minimize the field-of-view surrounding the cardiac tissue, minimize the slice thickness, and select appropriate reconstruction kernels for clinical indication and subject factors. Additional considerations regarding the cardiac phase should be accounted for to ensure the reconstructed images are free from artifacts across the coronaries.
The role of CCTA imaging for potential assessments in CAD
CCTA imaging plays a multifaceted role for the potential assessments in CAD. By extending the understanding of the three-dimensional anatomical structure of the heart and coronary arteries, the underlying physiological functional impacts of CAD can be assessed. As part of the CCTA imaging protocol, a non-contrast enhanced coronary calcium scan is typically performed to visualize any coronary calcifications which may serve as an indicator to proceed with the CCTA acquisition. The coronary calcification quantity is commonly assessed by the Agatston score method (4), which is widely used and accepted to determine coronary calcification.
Due to the nature of CCTA image acquisition, the technique is highly sensitive to detecting significant CAD stenosis at both a subject and vessel levels, as compared to invasive coronary angiography. The additional power of CCTA is based on the ability to identify non-obstructive CAD which is more challenging to detect with invasive angiography. By diagnosing individuals with non-obstructive CAD, treatment strategies can be employed earlier, potentially by clinical trial-based pharmaceutical intervention, and reduce the mortality risk associated with non-obstructive CAD.
Quantitative metrics describing the atherosclerotic plaque characteristics and imaging-based features can be derived from the CCTA images. Total plaque volume provides the quantity of plaque at both the subject and vessel levels, which is known to correlate with clinical outcomes. Furthermore, CCTA enables plaque feature characterizations based on the associated HU and visual patterns. High-risk plaque features from CCTA predict acute coronary syndrome events in addition to major adverse cardiovascular events (5). These CCTA-based high-risk plaque features are density-based plaque composition ranges (i.e., dense calcium, fibrous plaque, fibro-fatty plaque, necrotic core), low attenuation plaque, positive remodeling, napkin-ring sign, and spotty calcification. In addition to the plaque characterizations, a coronary inflammation image feature, known as the fat attenuation index, measures the perivascular adipose tissue HU, which has been shown to identify individuals at high risk for cardiac death and is suggested as method to guide early targeted primary prevention (6).
The extension of characterizing the anatomical structures corresponding to CAD is to determine the underlying functional and physiological consequence of CAD by assessing ischemia. In CCTA imaging, ischemia characterized using CT perfusion or non-invasive CT-derived fraction flow reserve (FFRCT). CT perfusion aids in detecting flow-limiting stenosis in patients with high coronary calcium scores or coronary stents. However, the technique has limitations associated with it, which restricts the clinical practicality. FFRCT provides a non-invasive assessment for ischemia through computational modeling of the coronary arteries based on standard CCTA acquisition. Therefore, the methodology can be retrospectively applied without any need for additional radiation exposure and associated CCTA image acquisition risks. FFRCT is effective and accurate in assessing ischemia and complements other CCTA based assessments. However, a significant limitation comes down to subject-specific image quality based on the CCTA acquisition. If the image acquisition generates a poor lumen delineation, then the FFRCT methodology breaks down.
Current and future methods to quantify atherosclerotic plaques
Current methods to quantify atherosclerotic plaques and their physiological impacts are primarily based on semi-automated processing techniques; however, more recently artificial intelligence (AI) techniques have emerged as next generation analysis tools, which reduce the need for manual inputs. Clinical adoption of these AI-based techniques is still in early phases, but some platforms have received regulatory approval for their applications to assess plaque quantity, as well as FFRCT. For many of these AI-based techniques, substantial effort demonstrating the superiority in plaque characterization and efficiency is still needed before integration into standard clinical workflows and clinical trial endpoint derivation, but the ability to demonstrate their power is reliant upon underlying CCTA image acquisition and overall image quality.
CCTA is positioned as a primary imaging modality for assessing CAD but is highly dependent on the image acquisition to ensure high-quality images are attained. Considerations must be taken with respect to the subject preparation steps, selection of image acquisition parameters and reconstruction parameters. These challenges for acquiring optimal images are even more important when considering qualitative and quantitative assessments for both structural and functional features generated from the image data. As imaging parameters directly influence the CCTA noise and HU, outcome assessments and image acquisition goals need to be considered prior to the scan. By considering the endpoint goals, high-quality images suitable for diagnostic purposes, as well as clinical trial assessments, are achievable for all imaging subjects.
Andrew Michalski, PhD, is a senior scientist and subject matter expert for cardio-thoracic computed tomography imaging at Calyx, a clinical research and regulatory solutions provider.
Rohit Sood MD, PhD, is the vice president and global head for cardio-thoracic imaging at Calyx. Calyx is a clinical research and regulatory solutions provider most relied on for solving complex challenges in clinical development.
References
1. Tsao, Connie W., et al. "Heart disease and stroke statistics—2023 update: a report from the American Heart Association." Circulation 147.8 (2023): e93-e621.
2. Abbara, Suhny, et al. "SCCT guidelines for the performance and acquisition of coronary computed tomographic angiography: A report of the society of Cardiovascular Computed Tomography Guidelines Committee: Endorsed by the North American Society for Cardiovascular Imaging (NASCI)." Journal of cardiovascular computed tomography 10.6 (2016): 435-449.
3. Knuuti, Juhani, et al. "2019 ESC Guidelines for the diagnosis and management of chronic coronary syndromes: the Task Force for the diagnosis and management of chronic coronary syndromes of the European Society of Cardiology (ESC)." European heart journal 41.3 (2020): 407-477.
4. Agatston, Arthur S., et al. "Quantification of coronary artery calcium using ultrafast computed tomography." Journal of the American college of cardiology 15.4 (1990): 827-832.
5. Shaw, Leslee J., et al. "Society of Cardiovascular Computed Tomography/North American Society of Cardiovascular Imaging–expert consensus document on coronary CT imaging of atherosclerotic plaque." Journal of Cardiovascular Computed Tomography 15.2 (2021): 93-109.
6. Antonopoulos, Alexios S., et al. "Detecting human coronary inflammation by imaging perivascular fat." Science translational medicine 9.398 (2017): eaal2658.
Related CCTA content
AI Breakthrough Award Presented to Cleerly CCTA Digital Care Platform
HeartFlow to Announce New Data at SCCT23
The Benefits of Coronary CTA in Pharmaceutical Clinical Trials
Photon-counting CT Noninvasively Detects Heart Disease in High-risk Patients
Rising Demand for Cardiac CT Positions Market for Major Growth